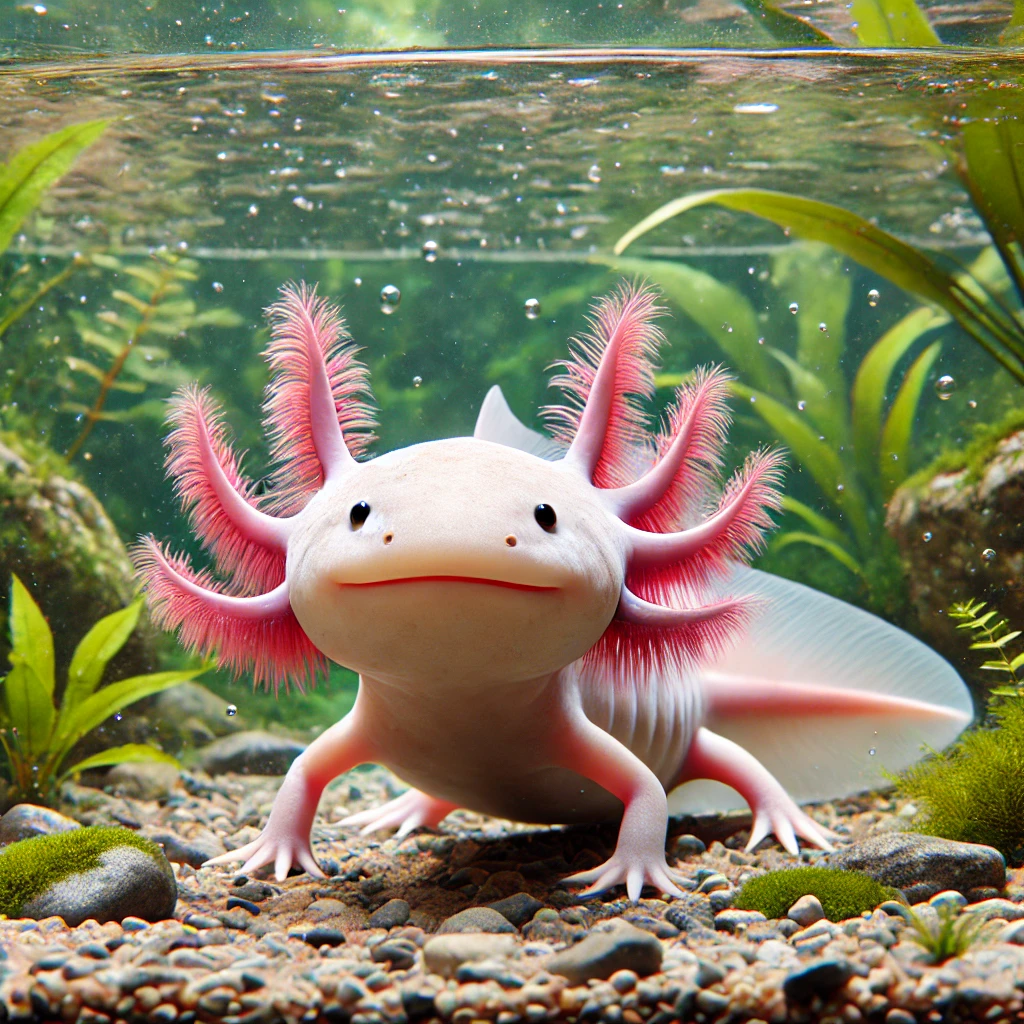
Unusual animals and their evolutionary characteristics (e.g., tardigrades, axolotls)
Welcome to a new journey to discover the wonders of our planet. Today, we will go beyond the beaten paths, where nature manifests itself in unusual and surprising forms. Let’s get ready to meet creatures that seem to defy the laws of biology and the true wonders of evolution and to discover the latest news from the scientific research that concerns them.
The Tardigrades: Tiny Giants of Survival
Imagine a living being capable of withstanding the most extreme temperatures, from outer space’s freezing stillness to boiling water’s infernal heat. A being who does not fear the void, radiation, or lack of water. It sounds like science fiction, yet it exists: it’s called a tardigrade, a tiny invertebrate that we can find everywhere, from the Himalayan peaks to the ocean depths.
Let’s observe it under the microscope: with its eight legs and stocky body, it resembles a little bear. But let’s not be fooled by appearances! This “water bear” is a true champion of endurance. His secret? Cryptobiosis is is a state of suspended life in which the metabolism slows to an almost complete stop. In this condition, the tardigrade can survive for decades, dehydrated, frozen, and bombarded by radiation. Think, friends, even a trip to space doesn’t scare him! And speaking of space, did you know that just this year, a team of scientists from the University of Florida sent tardigrades to the International Space Station to study how microgravity affects their survival ability? [Source: Phys.org] Who knows what secrets these incredible space travelers will reveal to us?
But how did the tardigrade acquire this extraordinary ability? Scientists believe it is a legacy of the origins of life when our planet was a far more hostile than today. Studying tardigrades could help us better understand the mechanisms of life and, who knows, develop new technologies for organ preservation or survival in extreme environments.
The Axolotl: Eternal Youth
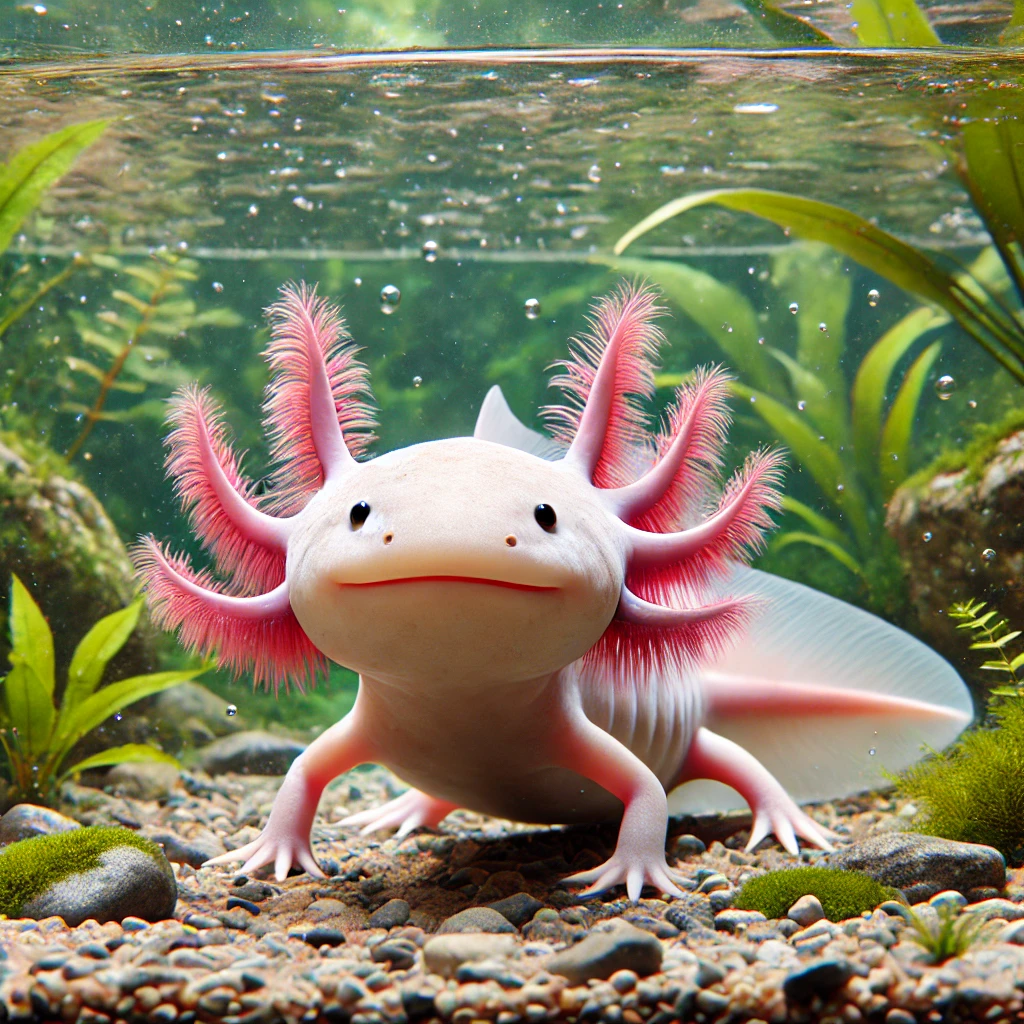
From the icy peaks and arid deserts, we now move to the waters of Mexico, where we meet an equally extraordinary creature: the axolotl. This salamander has a unique characteristic: neoteny, or the ability to retain its larval characteristics even into adulthood.
Look at its external, feather-like gills and long tail: they look like those of a tadpole, right? Yet, the axolotl is an adult animal capable of reproducing. This peculiarity is probably linked to the environment in which it lives, rich in water and poor in iodine, an essential element for metamorphosis.
But the axolotl has another surprising ability: regeneration. If he loses a limb, he regenerates it completely! Not only that, it can also regenerate parts of the spinal cord, heart, and eyes—a true miracle of nature, which fascinates scientists from all over the world. Recently, a group of researchers from the University of Kentucky made an incredible discovery: they identified a key gene in the axolotl’s regeneration process, opening up new perspectives for treating spinal injuries in humans. [Source: ScienceDaily] Understanding how the axolotl does all this could open up new avenues for regenerative medicine, offering hope to those who have suffered injuries or degenerative diseases.
Cave Fish: Adapting to the Darkness
We abandon the waters of Mexico and descend into the depths of the earth, into the dark and silent caves. Here live cave fish, pale and blind creatures, perfectly adapted to a world without light.
Think, friends, what it means to live in absolute darkness. The eyes become useless, an unnecessary burden. And so, in the course of evolution, cavefish lost them. But don’t think that they are helpless creatures! To orient themselves and hunt, they have developed other senses: touch, smell, and the ability to perceive the vibrations of water. A study published in “Nature” demonstrated that these fish have evolved a unique sensory system based on specialized cells that detect changes in water pressure, allowing them to “see” their surroundings even in the absence of light. [Source: Nature]
It is an extraordinary example of adaptation to the environment, a process that has shaped life on our planet for millions of years. What is most fascinating is that this phenomenon has been repeated in different parts of the world, in caves far from each other. The same environmental pressure, the lack of light, has led to similar solutions in different species, a phenomenon that scientists call evolutionary convergence.
The Grylloblattodea: Surviving the Frost
Let’s return to the surface, friends, and head towards the cold mountain regions. Here, we meet the Grylloblattodea, insects specialized in living in freezing temperatures. These little beings, similar to crickets without wings, are real “living icicles.”
Their metabolism is very slow, and they have adapted to extreme cold. They have lost the ability to react to heat, a price to pay for their specialization. But this strategy makes them vulnerable to climate change, a warning about the importance of preserving the balance of our planet. Unfortunately, recent studies have shown that some Grylloblattodea populations are declining due to global warming. [Source: National Geographic] We must protect these fragile mountain ecosystems quickly.
The Turtles: Resisting without Oxygen
Finally, friends, let’s return to the aquatic world to meet turtles, creatures that have developed another extraordinary ability: tolerance to anoxia, or a lack of oxygen.
Think of turtles living in lakes that freeze entirely in the winter. How do they survive without being able to breathe? Their secret is an anaerobic metabolism, which allows them to produce energy without oxygen. This is an incredible adaptation that could have distant roots in the evolutionary history of vertebrates. Did you know that a team of Chinese researchers recently discovered that turtles can survive for months in hypoxic conditions thanks to a mechanism that reduces brain activity and slows the heartbeat? [Source: BBC News] This discovery could have important implications for medicine, particularly for treating patients with brain damage caused by stroke or cardiac arrest.
A Heritage to be Protected
Friends, our journey through the wonders of evolution ends here. We met extraordinary creatures, each with its own story to tell. Tardigrades, axolotls, cavefish, Grylloblattodea, and turtles are just a few examples of our planet’s extraordinary biodiversity.
It is a precious heritage that we must protect and cherish for future generations. Studying these creatures does not only mean deepening our scientific knowledge but also learning to respect life in all its forms.
And with this, dear friends, I bid you farewell. I hope you are passionate about this journey and continue to explore the wonders of the world around us. Until next time!
Bibliographic references
- Avonce, N., Mendoza-Vargas, A., Morett, E., & Iturriaga, G. (2006). Insights on the evolution of trehalose biosynthesis. BMC Evolutionary Biology, 6(1), 109.
- Bickler, P. E., & Buck, L. T. (2007). Hypoxia tolerance in reptiles, amphibians, and fishes: life with variable oxygen availability. Annual Review of Physiology, 69(1), 145–170.
- Boothby, T. C., Goldstein, B. (2016). Reply to Bemm et al.: identification of foreign genes in 3 independent tardigrade genome assemblies. Proceedings of the National Academy of Sciences, 113(22), E3058-61.
- Boothby, T. C., Tenlen, J. R., Smith, F. W., Wang, J. R., Patanella, K. A., Osborne Nishimura, E., et al. (2015). Evidence for extensive horizontal gene transfer from the draft genome of a tardigrade. Proceedings of the National Academy of Sciences, 112(52), 15976–15981.
- Boschetti, C., Carr, A., Crisp, A., Eyres, I., Wang-Koh, Y., Lubzens, E., et al. (2012). Biochemical diversification through foreign gene expression in bdelloid rotifers. PLoS Genetics, 8(11), e1003035.
- Boschetti, C., Pouchkina-Stantcheva, N., Hoffmann, P. & Tunnacliffe, A. (2011). Foreign genes and novel hydrophilic protein genes participate in the desiccation response of the bdelloid rotifer Adineta ricciae. Journal of Experimental Biology, 214, 59–68.
- Bunngard, A., James, A. M., Gruszczyk, A. V., Martin, J., Murphy, M. P., & Fago, A. (2019). Metabolic adaptations during extreme anoxia in the turtle heart and their implications for ischemia-reperfusion injury. Scientific Reports, 9, 2850.
- Coppe, A., Agostini, C., Marino, I.A., Zane, L., Bargelloni, L., Bortoluzzi, S., & Patarnello, T. (2013). Genome evolution in the cold: Antarctic icefish muscle transcriptome reveals selective duplications increasing mitochondrial function. Genome Biology and Evolution, 5: 45–60.
- Ebersberger, I., Strauss, S., & von Haeseler, A. (2009). HAMSTr: Homology-Aware Analysis of Sequence Tags. Bioinformatics, 25(24), 3289-3291.
- Egginton, S., Skilbeck, C., Hoofd, L., Calvo, J., & Johnston, I. A. (2002). Peripheral oxygen transport in skeletal muscle of Antarctic and sub-Antarctic notothenioid fish. Journal of Experimental Biology, 205, 769–779.
- Evangelista, D., Schoville, S., Baccaro, F., & Ware, J. (2019) Anomalous Insect Wings: Unique Developmental Trajectories of Grylloblattodea Provide Insights into the Evolution of Insect Flight. Integrative and Comparative Biology. 59(2), 427-441.
- Gladyshev, E. A., & Arkhipova, I. R. (2007). Telomere-associated endonuclease-deficient Penelope-like retroelements in diverse eukaryotes. Proceedings of the National Academy of Sciences, 104(22), 9352–9357.
- Gladyshev, E. A., Meselson, M., & Arkhipova, I. R. (2008). Massive horizontal gene transfer in bdelloid rotifers. Science, 320(5880), 1210–1213.
- Graham, L. D., Blouin, J. L., & Davies, P. L. (2008). Type I antifreeze protein diversity in teleost fishes: A common microsatellite origin? BMC Evolutionary Biology, 8(1), 146.
- Gu, X., Fu, Y.-X., & Li, W.-H. (1995). Maximum likelihood estimation of the heterogeneity of substitution rate among nucleotide sites. Molecular Biology and Evolution, 12(4), 546–557.
- Haas, B. J., Papanicolaou, A., Yassour, M., Grabherr, M., Blood, P. D., Bowden, J., et al. (2013). De novo transcript sequence reconstruction from RNA-seq using the Trinity platform for reference generation and analysis. Nature Protocols, 8(8), 1494–1512.
- Hengherr, S., Worland, M. R., Reuner, A., Brümmer, F., & Schill, R. O. (2009). High-temperature tolerance in anhydrobiotic tardigrades is limited by glass transition. Physiological and Biochemical Zoology, 82(6), 749–755.
- Hespeels, B., Li, X., Flot, J-F., Pigneur, L-M., Malaisse, J., Da Silva, C., et al. (2015). Against all odds, trehalose-6-phosphate synthase and trehalase genes in the bdelloid rotifer adineta vaga were acquired by horizontal gene transfer and are upregulated during desiccation. PLoS ONE, 10(7), e0131313.
- Hochachka, P.W. (1986). Defense strategies against hypoxia and hypothermia. Science, 231, 234–241.
- Hofmann, G. E., Buckley, B. A., Airaksinen, S., Keen, J. E., & Somero, G. N. (2000). Heat-shock protein expression is absent in the Antarctic fish Trematomus bernacchii (family Nototheniidae). Journal of Experimental Biology, 203(15), 2331–2339.
- Hurvich, C. M., & Tsai, C.-L. (1989). Regression and time series model selection in small samples. Biometrics, 76(2), 297–307.
- Jombart, T., Kendall, B. E., Almagro-Garcia, J., & Colijn, C. (2017). TreeSpace: statistical exploration of phylogenetic tree sets. Bioinformatics, 33(16), 2547-2549.
- Jones, D. T., Taylor, W. R., & Thornton, J. M. (1992). The homology recognition potential of a sequence profile is enhanced by using sequences from structurally conserved regions of a protein family. Proteins, 13, 513–523.
- Kalyaanamoorthy , S. , Minh , B. Q. , Wong , T. K. F. , von Haeseler , A. , & Jermeen , L. S. (2017). ModelFinder: fast model selection for accurate phylogenetic estimates. Nature Methods, 14, 587–589.
- Katoh, K., & Standley, D. M. (2013). MAFFT multiple sequence alignment software version 7: Improvements in performance and usability. Molecular Biology and Evolution, 30(4), 772-780.
- Kosiol, C., & Goldman, N. (2005). Different amino-acid substitution models include different evolutionary information. Molecular Biology and Evolution, 22(9), 1934-1941.
- Kück, P., & Longo, G. C. (2014). FASconCAT-G: convenient sequence concatenation. Journal of Systematics and Evolution, 52(6), 687–689.
- Kück, P., Mayer, C., & Misof, B. (2010). AliScore: quantifying positional conservation in multiple sequence alignments. BMC Bioinformatics, 11, 573.
- Lande, R. (2009). Adaptation to an extraordinary environment by evolution of phenotypic plasticity and genetic assimilation. Journal of Evolutionary Biology, 22(7), 1435–1446.
- Langmead, B., & Salzberg, S. L. (2012). Fast gapped-read alignment with Bowtie 2. Nature Methods, 9(4), 357–359.
- Le, S. Q., & Gascuel, O. (2008). An improved general amino acid replacement matrix. Molecular Biology and Evolution, 25(7), 1307-1320.
- Le, S. Q., Dang, C., & Gascuel, O. (2012). Modeling protein evolution with several site-specific amino acid replacement matrices. Molecular Biology and Evolution, 29, 2921-2936.
- Lee, T. H., Guo, H., Wang, X., Kim, C., & Paterson, A. H. (2014). SNPhylo: a pipeline to construct a phylogenetic tree from huge SNP data. BMC Genomics, 15, 162.
- Li, B., & Dewey, C. N. (2011). RSEM: accurate transcript quantification from RNA-Seq data with or without a reference genome. BMC Bioinformatics, 12(1), 323.
- Liapis, A. I., Pim, M. L., & Bruttini, R. (1996). Research and development needs and opportunities in freeze drying. Drying Technology, 14(6), 1265–1300.
- Luu, B.E., & Storey, K.B. (2018). Solving donor organ shortage with insights from freeze tolerance in nature: activating endogenous antioxidant systems with non-coding RNA to precondition donor organs. Bioessays, 40(10), e1800092.
- Marks, C., Eme, J., Elsey, R.M., & Crossley, D.A. (2013). Chronic hypoxic incubation blunts thermally dependent cholinergic tone on the cardiovascular system in embryonic American alligator (Alligator mississippiensis). Journal of Comparative Physiology B, 183(7), 947–957.
- Michiels, C. (2004). Physiological and pathological responses to hypoxia. The American Journal of Pathology, 164(6), 1875–1882.
- Mirarab, S., & Warnow, T. (2015). ASTRAL-II: Coalescent-based species tree estimation with many hundreds of taxa and thousands of genes. Bioinformatics, 31(12), i44–i52.
- Misof, B., Liu, S., Meusemann, K., Peters, R.S., Donath, A., Mayer, C., et al. (2014). Phylogenomics resolves the timing and pattern of insect evolution. Science, 346(6210), 763-767.
- Müller, T., & Vingron, M. (2000). Modeling amino acid replacement. Journal of Computational Biology, 7(6), 761-776.
- Nguyen, L.-T., Schmidt, H. A., von Haeseler, A., & Minh, B. Q. (2015). IQ-TREE: a fast and effective stochastic algorithm for estimating maximum-likelihood phylogenies. Molecular Biology and Evolution, 32(1), 268-274.
- Petersen, M., Gahr, M., Mayer, C., Donath, A., von Reumont, B. M., & Misof, B. (2017). Orthograph: fast and accurate detection of orthologous groups in large datasets. Bioinformatics, 33(16), 2567-2569.
- Place, S. P., & Hofmann, G. E. (2005). Constitutive expression of a stress-inducible heat shock protein gene, hsp70, in phylogenetically distant Antarctic fish. Polar Biology, 28(4), 261–267.
- Predel, R., Neupert, S., Huetteroth, W., Kahnt, J., Waidelich, D., & Roth, S. (2012). Peptidomics-based phylogeny and biogeography of Mantophasmatodea (Hexapoda). Systematic Biology, 61(4), 609–629.
- R Core Team. (2016). R: A language and environment for statistical computing.
- Rambaut, A., & Drummond, A. J. (2009). TRACER: MCMC trace analysis tool version v1.5.0. Oxford University of Oxford.
- Rinehart, J. P., Hayward, S. A., Elnitsky, M. A., Sandro, L. H., Lee, R. E., & Denlinger, D. L. (2006). Continuous up-regulation of heat shock proteins in larvae, but not adults, of a polar insect. Proceedings of the National Academy of Sciences, 103(38), 14223–14227.
- Rizzello, A., Romano, A., Kottra, G., Acierno, R., Storelli, C., Verri, T., … Maffia, M. (2013). Protein cold adaptation strategy via a unique seven-amino acid domain in the icefish (Chionodraco hamatus) PEPT1 transporter. Proceedings of the National Academy of Sciences, 110(17), 7068–7073.
- Robertson, H. M. (2019). Molecular evolution of the major arthropod chemoreceptor gene families. Annual Review of Entomology, 64, 227–242.
- Robinson, M. D., McCarthy, D. J., & Smyth, G. K. (2009). edgeR: a Bioconductor package for differential expression analysis of digital gene expression data. Bioinformatics, 26(1), 139–140.
- Robinson, M. D. & Oshlack, A. (2010). A scaling normalization method for differential expression analysis of RNA-seq data. Genome Biology, 11(3), R25.
- Sakurai, M., Furuki, T., Akao, K., Tanaka, D., Nakahara, Y., Kikawada, T., et al. (2008). Vitrification is essential for anhydrobiosis in an African chironomid, Polypedilum vanderplanki. Proceedings of the National Academy of Sciences, 105(13), 5093–5098.
- Schoville, S. D., Simon, S., Bai, M., et al. (2021). Comparative transcriptomics of ice-crawlers demonstrates cold specialization constrains niche evolution in a relict lineage. Evolutionary Applications, 14, 360–382.
- Seppey, M., Manni, M. & Zdobnov, E. M. (2019). Gene prediction. Methods in Molecular Biology, 1962, 227-245.
- Sonnhammer, E. L. L., Eddy, S. R., & Durbin, R. (1997). Pfam: A comprehensive database of protein domain families based on seed alignments. Proteins, 28(3), 405–420.
- Soubrier, J., Galtier, N., Rodrigo, A., & Huchon, D. (2012). Less is more: How to choose the best substitution model for ancestral reconstruction in phylogenetics. Systematic Biology, 61, 655-670.
- Tapia, H., & Koshland, D. E. (2014). Trehalose is a versatile and long-lived chaperone for desiccation tolerance. Current Biology, 24(23), 2758–2766.
- The UniProt Consortium. (2019). UniProt: a worldwide hub of protein knowledge. Nucleic Acids Research, 47(D1), D506-D515.
- Udaka, H., Ueda, C., & Goto, S. G. (2010). Survival rate and expression of Heat-shock protein 70 and Frost genes after temperature stress in Drosophila melanogaster lines that are selected for recovery time from temperature coma. Journal of Insect Physiology, 56(12), 1889–1894.
- Veerassamy, S., Smith, A. B., & Tillier, E. R. M. (2003). A critical assessment of the potential of amino acid substitution models to adequately reflect the complex evolutionary process. Molecular Biology and Evolution, 20, 1406-1421.
- Whelan, S., & Goldman, N. (2001). A general empirical model of protein evolution derived from multiple protein families using a maximum-likelihood approach. Molecular Biology and Evolution, 18(5), 691-699.
- Wharton, D. A. (2002). Life at the limits: organisms in extreme environments. Cambridge: Cambridge University Press.
- Xie, Y., Wu, G., Tang, J., Luo, R., Patterson, J., Liu, S., … Li, Y. (2014). SOAPdenovo-Trans: de novo transcriptome assembly with the SOAPdenovo platform. GigaScience, 3(1), 18.
- Yang, Z. (1993). Maximum-likelihood estimation of phylogenetic trees from DNA sequences: a tutorial review. Molecular Biology and Evolution, 10(6), 1396-1401.
- Yoshida, M., Matsuda, H., Kubo, H., & Nishimura, T. (2016). Molecular characterization of Tps1 and Treh genes in Drosophila and their role in body water homeostasis. Scientific Reports, 6, 30582.
- Young, M. D., Wakefield, M. J., Smyth, G. K., & Oshlack, A. (2010). Gene ontology analysis for RNA-seq: accounting for selection bias. Genome Biology, 11(2), R14.
- Zatsepina, O., Przhiboro, A., Yushenova, I., Shilova, V., Zelentsova, E., Shostak, N., … Garbuz, D. (2016). A Drosophila heat shock response represents an exception rather than a rule among Diptera species. Insect Molecular Biology, 25(4), 431–449.