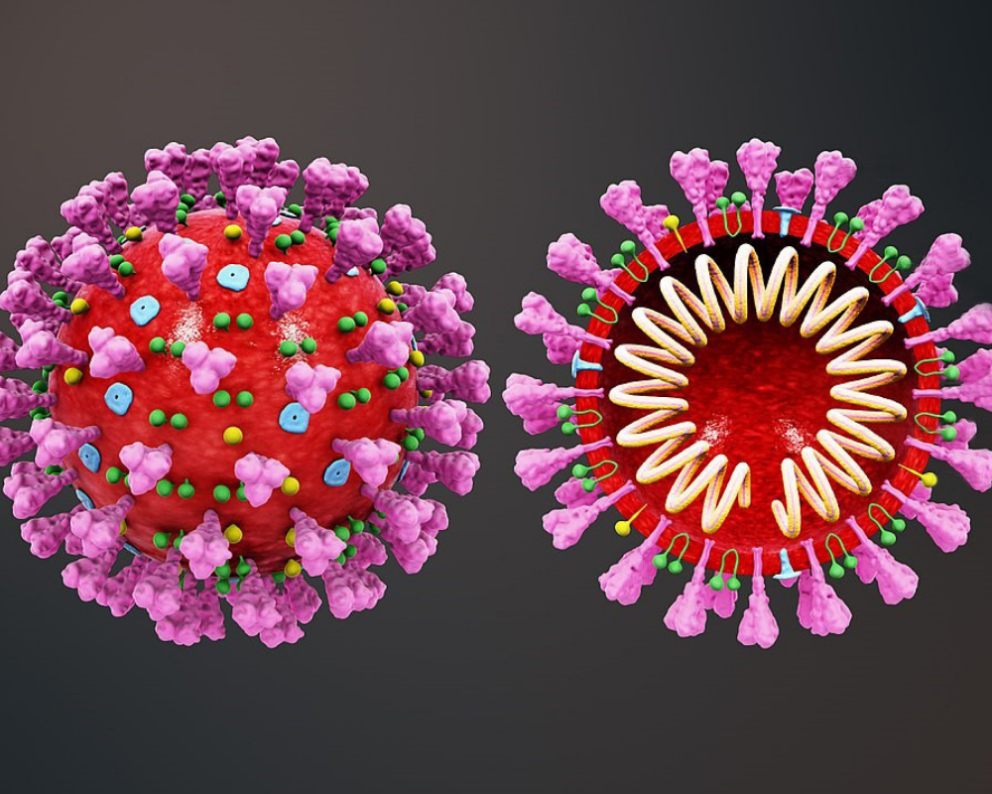
Imagine a world where even the most difficult diseases can be tackled with a completely new approach. No longer just traditional drugs or invasive interventions, but tiny biological factories, created in the laboratory, that work inside our bodies to repair, heal and protect. This is a reality that is emerging thanks to synthetic biology , a field of study that is redefining the boundaries of medicine.
Synthetic biology is like the engineering of life. Researchers, using knowledge from molecular biology, design and build artificial biological systems, such as synthetic cells , with specific functions. These cells are not “living” in the traditional sense, but they are powerful tools, capable of performing tasks that natural cells cannot do or do less efficiently.
A bottom-up approach: building artificial cells
The process of creating an artificial cell is like a building block, but at the molecular level. It starts with basic biological components, such as lipids, proteins, nucleic acids, and assembles them in a controlled way to create a structure that mimics a cell. This approach, called “bottom-up,” offers great flexibility, allowing cells to be designed with customized characteristics.
There are two main strategies for creating artificial cells:
- Top-Down Approach : Starting from an existing cell, simplifying it and removing components that are not essential for the desired function.
- Bottom-Up Approach : The cell is built from scratch, assembling biological or synthetic molecules. Artificial cells created with the bottom-up approach do not have an autonomous control system, like natural cells, but are completely controlled by the designer. This allows their behavior to be precisely programmed, like a biological micro-robot.
Why are artificial cells important?
You may ask, “Why do we need artificial cells? Aren’t natural cells enough?” The answer is that artificial cells offer unique advantages.
- Efficiency: Artificial cells can be designed to perform a specific function without “distractions” or unwanted interactions, unlike natural cells, which perform multiple functions simultaneously.
- Safety: Artificial cells, being non-living, reduce the biosafety concerns associated with the use of living organisms. There is no risk of uncontrolled release into the environment.
- Versatility: Synthetic biology allows the creation of artificial cells with unique characteristics by combining biological and synthetic elements, opening up a world of possibilities.
Medical applications
Artificial cells have enormous potential in the medical field, with applications ranging from diagnosis to therapy. Let’s see some concrete examples:
- Targeted drug delivery: Artificial cells can be used as “smart carriers” to deliver drugs precisely, directly to the site of disease. Imagine a microscopic capsule that travels through the body, recognizes tumor cells, and releases the chemotherapy drug only there, sparing healthy cells. This reduces side effects and increases the effectiveness of the treatment. Liposomes, vesicles composed of lipids, are often used as the casing for these drug deliveries.
- Biosensors: Artificial cells can be engineered to detect specific disease biomarkers, such as proteins or nucleic acids that are present in abnormal amounts in a patient. These biosensors can provide early and accurate diagnosis, even at the molecular level. In this case, artificial cells can be “programmed” with proteins that react to a given signal in the environment.
- Therapeutic Protein Production: Artificial cells can act as small factories to produce therapeutic proteins, such as antibodies or enzymes, directly in the patient’s body. This application could be particularly useful for treating genetic or rare diseases, where defective proteins need to be replaced.
- Gene therapy: Artificial cells can deliver genetic material (DNA or RNA) into a patient’s cells to correct genetic defects. This application offers a promising approach to treating inherited diseases or cancers.
- Artificial Photosynthesis: Some artificial cells are capable of photosynthesis, using light to produce energy and useful compounds. These types of cells could be used to generate energy within the body or to produce drugs sustainably.
- Immunotherapy: Artificial cells can be used to stimulate a patient’s immune system to recognize and destroy tumor cells. Artificial cells can be programmed to synthesize therapeutic proteins within tumors. They can also mimic immune cells to target therapy.
- Models for Research: Artificial cells offer a simplified model for the study of cellular processes, particularly in origin-of-life research and theoretical biology.
Other applications
The applications of synthetic biology are not limited to medicine. Artificial cells can be used in many other areas, including:
- Industrial Biotechnology: Artificial cells can produce chemical compounds, biofuels, or innovative materials more efficiently and sustainably than traditional methods.
- Environmental Biosensors: Artificial cells can detect pollutants in the environment, providing an early warning system to protect human health and the ecosystem.
- Smart Materials: Artificial cells can be used to create materials with surprising properties, such as the ability to self-repair or adapt to their surroundings.
Future prospects
Synthetic biology is a rapidly evolving field with great promise, but also significant challenges.
- Complexity: The construction of complex artificial cells, with multiple integrated functions, is a difficult task that requires a multidisciplinary approach and new technologies.
- Control: We need to find ways to control the behavior of artificial cells within the body, ensuring that they perform their functions safely and predictably.
- Scalability: Methods need to be developed to produce artificial cells in large quantities and at low costs to make them accessible to all.
- Theoretical foundations: Synthetic biology needs further developments in its theoretical foundations to be able to advance rapidly in the development of increasingly complex and more autonomous systems.
- Ethical Aspects: It is important to reflect on the ethical aspects related to the creation and use of artificial biological systems.
Despite these challenges, the future of synthetic biology is bright. Researchers are working enthusiastically to overcome the difficulties and realize the full potential of this technology. In the future, artificial cells could become a fundamental part of our lives, improving our health and our environment.
Synthetic Biology in the News
Synthetic biology is making significant progress, with recent developments highlighting its potential in medicine and biotechnology.
Photosynthetic animal cells
A team of scientists has successfully introduced chloroplasts, the organelles responsible for photosynthesis in plants, into animal cells. Using chloroplasts isolated from the red algae Cyanidioschyzon merolae, which can photosynthesize at temperatures above 37°C, they were able to integrate them into Chinese hamster ovary cells. These cells showed photosynthetic activity for several days, accelerating their growth. Although the imported chloroplasts degraded by day four, this achievement opens the door to creating artificially photosynthetic animal cells, with potential applications in the production of artificial organs and lab-grown meat.
Synthetic genes for tissue construction
Researchers at the University of Rome Tor Vergata and the University of California, Los Angeles have developed synthetic genes capable of replicating the activity of cells in the construction and dismantling of molecular structures. Using synthetic DNA “building blocks,” they created tubular structures controlled by specific RNA sequences, allowing the structures to form or disassemble at precise times. This approach could lead to new applications in biomedicine and diagnostics, enabling the design of materials that evolve spontaneously over time.
Communication between synthetic and natural cells
Another advance involves the creation of synthetic cells capable of communicating with natural cells. These synthetic cells, equipped with artificial organelles, can respond to environmental stimuli and exchange signals with biological cells. This communication ability could revolutionize regenerative medicine and the creation of hybrid tissues, combining natural and synthetic elements to treat different pathologies.
These developments underscore the rapid advances of synthetic biology and its potential to transform therapeutic and biotechnological approaches.
Key Points to Remember:
- Synthetic biology is a field that aims to build artificial biological systems with specific functions.
- Artificial cells are powerful tools for medicine and biotechnology.
- They can be designed with unique features through a “bottom-up” approach.
- They have potential applications in various fields, such as therapy, diagnosis, and materials production.
- Research in this field is advancing rapidly, but it is important to address the challenges with an ethical and responsible approach.
- Artificial cells are complex systems, but at the same time simplified compared to natural cells and can be used as models for the study of theoretical biology and the origin of life.
Test Your Knowledge on Synthetic Biology!
Answer the following questions to check your understanding of the article on synthetic biology.
Bibliographic references
- Adamala, Katarzyna P., Martin-Alarcon, Daniel A., Guthrie-Honea, Katriona R., and Boyden, Edward S. 2017. “Engineering Genetic Circuit Interactions within and between Synthetic Minimal Cells.” Nature Chemistry 9 (5): 431–439
- Adiga, Rajani, Al-Adhami, Mustafa, Andar, Abhay, Borhani, Shayan, Brown, Sheniqua, Burgenson, David, Cooper, Merideth A., et al. 2018. “Point-of-Care Production of Therapeutic Proteins of Good-Manufacturing-Practice Quality.” Nature Biomedical Engineering 2 (9): 675–686
- Adiga, Rajani, Andar, Abhay, Borhani, Shayan, Burgenson, David, Deldari, Sevda, Frey, Douglas, Ge, Xudong, et al. 2020. “Manufacturing Biological Medicines on Demand: Safety and Efficacy of Granulocyte Colony-stimulating Factor in a Mouse Model of Total Body Irradiation.” Biotechnology Progress
- Aghdam Abri, Marjan Roya Bagheri, Mosafer Jafar, Baradaran Behzad, Hashemzaei Mahmoud, Baghbanzadeh Amir, de la Guardia Miguel, and Mokhtarzadeh Ahad. 2019. “Recent Advances on Thermosensitive and pH-Sensitive Liposomes Employed in Controlled Release.” Journal of Controlled Release: Official Journal of the Controlled Release Society 315 (December): 1–22
- Aimon, Sophie, Manzi, John, Schmidt, Daniel, Jose Antonio Poveda Larrosa, Patricia Bassereau, and Toombes Gilman E. S. 2011. “Functional Reconstitution of a Voltage-Gated Potassium Channel in Giant Unilamellar Vesicles.” PLoS One 6 (10): e25529
- Allen, Theresa M., and Cullis, Pieter R. 2013. “Liposomal Drug Delivery Systems: From Concept to Clinical Applications.” Advanced Drug Delivery Reviews 65 (1): 36–48
- Altamura, Emiliano; Albanese, Paola; Marotta, Roberto; Milano, Francesco; Fiore, Michele; Trotta, Massimo; Stano, Pasquale; and Mavelli, Fabio. nd “Light-Driven ATP Production Promotes mRNA Biosynthesis inside Hybrid Multi-Compartment Artificial Protocells.”
- Altamura, Emiliano, Carrara, P., D’Angelo, F., Mavelli, F., and Stano, P. 2018. “Extrinsic stochastic factors (solute partition) in gene expression inside lipid vesicles and lipid-stabilized water-in-oil droplets: A review.” Synth. Biol. 3
- Amos, M., Dittrich, P., McCaskill, J., and Rasmussen, S. 2011. “Biological and Chemical Information Technologies.” Procedia Comput. Sci. 7, 56–60
- Amstad, Esther, Kohlbrecher, Joachim, Müller, Elisabeth, Schweizer, Thomas, Textor, Marcus, and Reimhult, Erik. 2011. “Triggered Release from Liposomes through Magnetic Actuation of Iron Oxide Nanoparticle Containing Membranes.” Nano Letters 11 (4): 1664–1670
- Anta, J. 2021. “Information, meaning and physics: The intellectual evolution of the English School of Information Theory during 1946-1956.” Sci. Context 34, 357–373
- Ashby, W.R. 1956. An Introduction to Cybernetics; Chapman & Hall Ltd.: London, UK
- Ashby, W.R. 1960. Design for a Brain, 2nd ed.; John Wiley & Sons Inc.: New York, NY, USA
- Ashkenasy, G., Hermans, T.M., Otto, S., and Taylor, A.F. 2017. “Systems chemistry.” Chem. Soc. Rev. 46, 2543–2554
- Atlan, H. 1977. “Sources of Information in Biological Systems.” IFAC Proc. Vol. 10, 177–184
- Atlan, H. 1987. “Self Creation of Meaning.” Phys. Scr. 36, 563–576
- “Activation of the Bacterial Sensor Kinase PhoQ by Acidic pH.” 2007. Molecular Cell 26 (2): 165–174
- Baimanov, Cai, and Chen 2019.
- Baker, BM, Trappmann, B, Wang, WY, Sakar, MS, Kim, IL, Shenoy, VB, Burdick, JA, & Chen, CS 2015, Cell-mediated fibre recruitment drives extracellular matrix mechanosensing in engineered fibrillar microenvironments. Nat. Mater. 14, 1262–1268
- Ball, P. 2005. “Synthetic biology for nanotechnology.” Nanotechnology 16, R1–R8
- Bangham, AD, et al. 1965. “Diffusion of univalent ions across lamellae of swollen phospholipids.” J. Mol. Biol. 13, 238
- Basu, S, et al. 2004. “Spatiotemporal control of gene expression with pulse-generating networks.” Proc. Natl. Acad. Sci. U.S.A. 101, 6355–6360
- Bath, J, et al. 2005. “A free-running DNA motor powered by a nicking enzyme.” Angew. Chem., Int. Ed. 44, 4358–4361
- Baumann , Andreas , Brändli-Baiocco , Annamaria , Cavagnaro , Joy , Dempster , Maggie , Depelchin , B Olympe , Ivens , Inge A. , et al. 2014. “PEGylated Biopharmaceuticals: Current Experience and Considerations for Nonclinical Development.” Toxicologic Pathology 43(7):959–983
- Beal, J & Rogers, M. 2020. “Levels of autonomy in synthetic biology engineering.” Mol. Syst. Biol. 16, e10019
- Bedau, M.A. 2003. “Artificial life: Organization, adaptation and complexity from the bottom up.” Trends Cogn. Sci. 7, 505–512
- Bedau, M.A., McCaskill, J.S., Packard, N.H., and Rasmussen, S. 2010. “Living technology: Exploiting life’s principles in technology.” Artif. Life 16, 89–97
- Berhanu, Samuel, Ueda, Takuya, and Kuruma, Yutetsu. 2019. “Artificial Photosynthetic Cell Producing Energy for Protein Synthesis.” Nature Communications 10 (1): 1325
- Bertschinger, N., Olbrich, E., Ay, N., and Jost, J. 2008. “Autonomy: An information theoretic perspective.” Biosystems 91, 331–345
- Biner, Olivier, Fedor, Justin G., Yin, Zhan, and Hirst, Judy. 2020. “Bottom-Up Construction of a Minimal System for Cellular Respiration and Energy Regeneration.” ACS Synthetic Biology 9 (6): 1450–1459
- Blake, WJ, et al. 2003. “Noise in eukaryotic gene expression.” Nature (London) 422, 633–637
- Bloch, J, Bachoud-Lévi, AC, Déglon, N, Lefaucheur, JP, Winkel, L, Palfi, S, Nguyen, JP, et al. 2004. “Neuroprotective Gene Therapy for Huntington’s Disease, Using Polymer-Encapsulated Cells Engineered to Secrete Human Ciliary Neurotrophic Factor: Results of a Phase I Study.” Human Gene Therapy 15 (10): 968–975
- Boyer, Cecile, and Zasadzinski, Joseph A. 2007. “Multiple Lipid Compartments Slow Vesicle Contents Release in Lipases and Serum.” ACS Nano 1 (3): 176–182
- Braccini, M., Collinson, E., Roli, A., Fellermann, H., and Stano, P. 2023. “Recurrent neural networks in synthetic cells: a route to autonomous molecular agents?” Front. Bioeng. Biotechnol. 11, 1210334
- Brillouin, L. 1962. Science and Information Theory, 2nd ed.; Academic Press, Inc.: New York, NY, USA
- Buxboim, A, et al. 2007. “A single-step photolithographic interface for cell-free gene expression and active biochips.” Small 3, 500–510
- Cariani, P. 1993. “To evolve an ear. Epistemological implications of Gordon Pask’s electrochemical devices.” Syst. Res. 10, 19–33
- Carnap, R., and Bar-Hillel, Y. 1953. “An outline of a theory of semantic information.” Brit. J. Phil. Sci. 4, 147–157
- Carter, Kevin A., Shao, Shuai, Hoopes, Matthew I., Luo, Dandan, Ahsan, Bilal, Grigoryants, Vladimir M., Song, Wentao, et al. 2014. “Porphyrin–phospholipid Liposomes Permeabilized by near-Infrared Light.” Nature Communications
- Caschera, Filippo, and Noireaux, Vincent. 2015. “A Cost-Effective Polyphosphate-Based Metabolism Fuels an All E. Coli Cell-Free Expression System.” Metabolic Engineering
- Cattaneo, MV, and Chang, TM. 1991. “The Potential of a Microencapsulated Urease-Zeolite Oral Sorbent for the Removal of Urea in Uremia.” ASAIO Transactions / American Society for Artificial Internal Organs 37 (2): 80–87
- Čejková, J., and Cartwright, J.H.E. 2022. “Chemobrionics and Systems Chemistry.” ChemSystemsChem 4, e202200002
- “Cell-Free Expression and Assembly of ATP Synthase.” 2011. Journal of Molecular Biology 413 (3): 593–603
- Center for Drug Evaluation, and Research. 2019. “Emerging Technology Program.” October 10, 2019.
- Chang, Hao-Nan, Liu, Bei-Yuan, Qi, Yun-Kun, Zhou, Yang, Chen, Yan-Ping, Pan, Kai-Mai, Li, Wen-Wen, et al. 2015. “Blocking of the PD-1/PD-L1 Interaction by a D-Peptide Antagonist for Cancer Immunotherapy.” Applied Chemistry 54 (40): 11760–11764
- Chang, T.M. 1964. “Semipermeable microcapsules.” Science 146, 524–525
- Chang, T.M. 1967. “Microcapsules as artificial cells.” Sci. J. 3, 62
- Chang, T.M. 1972. Artificial Cells; Charles C. Thomas: Springfield, IL, USA
- Chang, T.M. 2000. “Artificial cell biotechnology for medical applications.” Blood Purif. 18, 91–96
- Chang, Thomas Ming Swi. 2005. “Therapeutic Applications of Polymeric Artificial Cells.” Nature Reviews Drug Discovery
- Chang, Thomas Ming Swi. 2019. “ARTIFICIAL CELL Evolves into Nanomedicine, Biotherapeutics, Blood Substitutes, Drug Delivery, Enzyme/gene Therapy, Cancer Therapy, Cell/stem Cell Therapy, Nanoparticles, Liposomes, Bioencapsulation, Replicating Synthetic Cells, Cell Encapsulation/ scaffold, Biosorbent/immunosorbent Haemoperfusion/plasmapheresis, Regenerative Medicine, Encapsulated Microbe, Nanobiotechnology, Nanotechnology.” Artificial Cells, Nanomedicine, and Biotechnology 47 (1): 997–1013
- Chen, JH, and Seeman, NC. 1991. “Synthesis from DNA of a molecule with the connectivity of a cube.” Nature (London) 350, 631–633
- Chi, Yingying, Yin, Xuelei, Sun, Kaoxiang, Feng, Shuaishuai, Liu, Jinhu, Chen, Daquan, Guo, Chuanyou, and Wu, Zimei. 2017. “Redox-Sensitive and Hyaluronic Acid Functionalized Liposomes for Cytoplasmic Drug Delivery to Osteosarcoma in Animal Models.” Journal of Controlled Release: Official Journal of the Controlled Release Society 261 (September): 113–125
- Chin, JW. 2006. “Modular approaches to expanding the functions of living matter.” Nat. Chem. Biol. 2, 304–311
- Choi, Hyo-Jick, and Montemagno, Carlo D. 2005. “Artificial Organelle: ATP Synthesis from Cellular Mimetic Polymersomes.” Nano Letters
- Chworos, A, et al. 2004. “Building programmable jigsaw puzzles with RNA.” Science 306, 2068–2072
- Cronin, L., Krasnogor, N., Davis, B.G., Alexander, C., Robertson, N., Steinke, J.H.G., Schroeder, S.L.M., Khlobystov, A.N., Cooper, G., Gardner, P.M., et al. 2006. “The imitation game–a computational chemical approach to recognizing life.” Nat. Biotechnol. 24, 1203–1206
- Damiano, L., and Stano, P. 2018. “Synthetic Biology and Artificial Intelligence. Grounding a cross-disciplinary approach to the synthetic exploration of (embodied) cognition.” Complex Syst. 27, 199–228
- Damiano, L., and Stano, P. 2020. “On the “Life-Likeness” of Synthetic Cells.” Front. Bioeng. Biotechnol. 8, 953
- Damiano, L., and Stano, P. 2021. “A Wetware Embodied AI? Towards an Autopoietic Organizational Approach Grounded in Synthetic Biology.” Front. Bioeng. Biotechnol. 9, 873
- Damiano, L., and Stano, P. 2023. “Explorative Synthetic Biology in AI. Criteria of relevance and a taxonomy for synthetic models of living and cognitive processes.” Artif. Life 29, 367–387
- Danhier 2016.
- de Almeida, P, Jaspers, M, Vaessen, S, Tagit, O, Portale, G, Rowan, AE & Kouwer, PHJ. 2019. “Cytoskeletal Stiffening in Synthetic Hydrogels.” Nat. Common. 10, 609
- de Lorenzo, V., and Danchin, A. 2008. “Synthetic biology: discovering new worlds and new words.” EMBO Rep. 9, 822–827
- Del Moro, L., Magarini, M., and Stano, P. 2024. “On the Evaluation of Observed Semantic Information in Synthetic Cells.” In Proceedings of the Artificial Life and Evolutionary Computation, WIVACE 2023, Venice, Italy, 6–8 September 2023; Communications in Computer and Information Science; Serra, R., Villani, M., Cagnoni, S., Eds.; Springer Nature Switzerland: Cham, Switzerland
- Del Moro, L., Ruzzante, B., Magarini, M., Gentili, P.L., Rampioni, G., Roli, A., Damiano, L., and Stano, P. 2023. “Chemical Neural Networks and Semantic Information Investigated Through Synthetic Cells.” In Proceedings of the Artificial Life and Evolutionary Computation, WIVACE 2022, Gaeta, Italy, 14–16 September 2022; Communications in Computer and Information Science; De Stefano, C., Fontanella, F., Vanneschi, L., Eds.; Springer Nature Switzerland: Cham, Switzerland
- Deplazes-Zemp, A. 2016. “Artificial cell research as a field that connects chemical, biological and philosophical questions.” Chimia 70, 443–448
- Deshpande, Siddharth, Caspi, Yaron, Meijering, Anna E. C., and Dekker, Cees. 2016. “Octanol-Assisted Liposome Assembly on Chip.” Nature Communications 7 (January): 10447
- Deshpande, Siddharth, Spoelstra, Willem Kasper, van Doorn, Marleen, Kerssemakers, Jacob, and Dekker, Cees. 2018. “Mechanical Division of Cell-Sized Liposomes.” ACS Nano 12 (3): 2560–2568
- Dhir, Satarupa, Salahub, Sumalee, Anu, Stella Mathews, Surjith Kumar Kumar, Montemagno, Carlo D., and Abraham, Sinoj. 2018. “Light-Induced ATP Driven Self-Assembly of Actin and Heavy-Meromyosin in Proteo-Tubularsomes as a Step toward Artificial Cells.” Chemical Communications 54(42):5346–5349
- Di Paolo, E.A. 2003. “Organismically inspired robotics: Homeostatic adaptation and teleology beyond the closed sensorimotor loop.” In Dynamical Systems Approach to Embodiment and Sociality; Murase, K., Asakura, T., Eds.; Advanced Knowledge International: Adelaide, Australia, pp. 19–42
- http://dx.doi.org/10.1037/0033-295X.102.2.205 Dimova, R., Stano, P., Marques, C. C., and Walde, P. 2020 . In The Giant Vesicle Book ; Dimova , R. , Marques , CM , Eds.; Taylor & Francis Group: Boca Raton, FL, USA, pp. 10-11. 3–20
- Ding, Yudi, Williams, Nicholas H., and Hunter, Christopher A. 2019. “A Synthetic Vesicle-to-Vesicle Communication System.” Journal of the American Chemical Society 141 (44): 17847–17853
- Dittrich, Petra S., Heule, Martin, Renaud, Philippe, and Manz, Andreas. 2006. “On-Chip Extrusion of Lipid Vesicles and Tubes through Microsized Apertures.” Lab on a Chip 6 (4): 488–493
- Doktorova, Milka, Heberle, Frederick A., Eicher, Barbara, Standaert, Robert F., Katsaras, John, London, Erwin, Pabst, Georg, and Marquardt, Drew. 2018. “Preparation of Asymmetric Phospholipid Vesicles for Use as Cell Membrane Models.” Nature Protocols 13 (9): 2086–2101
- Fatouros, Dimitrios G., Lamprou, Dimitrios A., Urquhart, Andrew J., Yannopoulos, Spyros N., Vizirianakis, Ioannis S., Zhang, Shuguang, and Koutsopoulos, Sotirios. 2014. “Lipid-like Self-Assembling Peptide Nanovesicles for Drug Delivery.” ACS Applied Materials & Interfaces 6 (11): 8184–8189
- Fang, Islam, and Maeda 2020.
- Feng, Shuaishuai, Wu, Zi-Xin, Zhao, Ziyan, Liu, Jinhu, Sun, Kaoxiang, Guo, Chuanyou, Wang, Hongbo, and Wu, Zimei. 2019. “Engineering of Bone- and CD44-Dual-Targeting Redox-Sensitive Liposomes for the Treatment of Orthotopic Osteosarcoma.” ACS Applied Materials & Interfaces 11 (7): 7357–7368
- Feng, Xiyun, Jia, Yi, Cai, Peng, Fei, Jinbo, and Li, Junbai. 2016. “Coassembly of Photosystem II and ATPase as Artificial Chloroplast for Light-Driven ATP Synthesis.” ACS Nano 10 (1): 556–561
- Ferreira, SA, Motwani, MS, Faull, PA, Seymour, AJ, Yu, TTL, Enayati, M, Taheem, DK, Salzlechner, C, Haghighi, T, Kania, EM, Oommen, OP, Ahmed, T, Loaiza, S, Parzych, K, Dazzi, F, Varghese, FOP, Grigosty, A.E. Auner, HW, Snijders, AP, Bozec, L & Gentleman, E. 2018. “Bi-directional cell-pericellular matrix interactions direct stem cell fate.” Nat. Common. 9
- Fields, C., and Levin, M. 2020. “How Do Living Systems Create Meaning?” Philosophies 5, 36
- Floridi, L. 2011. The Philosophy of Information; Oxford University Press: Oxford, UK
- Froese, T., and Ziemke, T. 2009. “Enactive artificial intelligence: Investigating the systemic organization of life and mind.” Artif. Intell. 173, 466–500
- Fujii, S., Matsuura, T., Sunami, T., Nishikawa, T., Kazuta, Y., and Yomo, T. 2014. “Liposome display for in vitro selection and evolution of membrane proteins.” Nat. Protoc. 9, 1578–1591
- Garenne, D, Libchaber, A & Noireaux, V. 2020. “Membrane molecular crowding enhances MreB polymerization to shape synthetic cells from spheres to rods.” Proc. Natl. Acad. Sci. U. S. A 117, 1902–1909
- Gardner, P.M., Winzer, K., and Davis, B.G. 2009. “Sugar synthesis in a protocellular model leads to a cell signalling response in bacteria.” Nat. Chem. 1, 377–383
- Gentili, P.L. 2013. “Small steps towards the development of chemical artificial intelligent systems.” RSC Adv. 3, 25523–25549
- Gentili, P.L., and Stano, P. 2022. “Chemical Neural Networks Inside Synthetic Cells? A Proposal for Their Realization and Modeling.” Front. Bioeng. Biotechnol. 10, 927110
- Giulimondi et al. 2019.
- Goodman, et al. 2005.
- Green, ML, Choi, CL, Hattrick-Simpers, JR, Joshi, AM, Takeuchi, I, Barron, SC, Campo, E, Chiang, T, Empedocles, S, Gregoire, JM, Kusne, AG, Martin, J, Mehta, A, Persson, K, Trautt, Z, Van Duren, J & Zakutayev, A. 2017. “Fulfilling the promise of the materials genome initiative with high-throughput experimental methodologies.” Appl. Phys. Rev. 4, 011105
- Grubbe, William S., Rasor, Blake J., Krüger, Antje, Jewett, Michael C., and Karim, Ashty S. 2020. “Cell-Free Styrene Biosynthesis at High Titers.” Metabolic Engineering 61 (September): 89–95
- Gu, Kang Fu, and Chang, Thomas Ming Swi. 1990. “Conversion of Ammonia or Urea into Essential Amino Acids, L-Leucine, L-Valine, and L-Isoleucine Using Artificial Cells Containing an Immobilized Multienzyme System and Dextran-NAD.” Journal of Molecular Catalysis
- Guidoni, A. 2018. Towards the Robot Sapiens , 1st ed.; Science Series, Controluce Editions: Monte Compatri, Italy
- Haller, Barbara, Kerstin Göpfrich, Martin Schröter, Janiesch Jan-Willi, Platzman Ilia, and Spatz, Joachim P. 2018. “Charge-Controlled Microfluidic Formation of Lipid-Based Single- and Multicompartment Systems.” Lab on a Chip 18 (17): 2665–2674
- Hanczyc, M.M. 2009. “The Early History of Protocells – The search for the recipe of life.” In Protocells: Bridging Nonliving and Living Matter; Rasmussen, S., Bedau, M.A., Chen, L., Deamer, D., Krakauer, D.C., Packard, N.H., Eds.; MIT Press: Cambridge MA, USA, pp. 3–18
- Harnad, S. 1990. “The symbol-grounding problem.” Phys. D Nonlinear Phenom. 42, 335–346
- Hayles, N.K. 1999. How We Became Posthuman. Virtual Bodies in Cybernetics, Literature, and Informatics; The University of Chicago Press: Chicago, IL, USA; London, UK
- He, Haisheng, Lu, Yi, Qi, Jianping, Zhu, Quangang, Chen, Zhongjian, and Wu, Wei. 2019. “Adapting Liposomes for Oral Drug Delivery.” Acta Pharmaceutica Sinica. B 9 (1): 36–48
- Hoffmann, P.M. 2012. Life’s Ratchet. How Molecular Machines Extract Order from Chaos, 1st ed.; Basic Books. A Member of the Perseus Books Group: New York, NY, USA
- Hörner, M, Raute, K, Hummel, B, Madl, J, Creusen, G, Thomas, OS, Christen, EH, Hotz, N, Gübeli, RJ, Engesser, R, Rebmann, B, Lauer, J, Rolauffs, B, Timmer, J, Schamel, WWA, Pruszak, J, Rümer, W, Zurbriggen, MD, Friedrich, C, Walther, A, Minguet, S, Sawarkar, R & Weber, W. 2019. “Phytochrome-Based Extracellular Matrix with Reversibly Tunable Mechanical Properties.” Adv. Mater 31, e1806727
- Hortelão, Ana C., Sonia García‐Jimeno Mary Cano‐Sarabia, Tania Patiño Daniel Maspoch, and Sanchez Samuel. 2020. “LipoBots: Using Liposomal Vesicles as Protective Shell of Urease‐Based Nanomotors.” Advanced Functional Materials
- Hu, Quanyin, Sun, Wujin, Qian, Chengen, Wang, Chao, Bomba, Hunter N., and Gu, Zhen. 2015. “Anticancer Platelet-Mimicking Nanovehicles.” Advanced Materials 27 (44): 7043–7050
- Hu, Yumiao, and Qiu, Liyan. 2019. “Polymersomes: Preparation and Characterization.” In Pharmaceutical Nanotechnology: Basic Protocols, edited by Weissig Volkmar and Elbayoumi Tamer, 247–265. New York, NY: Springer New York
- Hutchison, CA; Chuang, RY; Noskov, VN; Assad-Garcia , N. ; Deerinck , TJ ; Ellisman , MH ; Gill , J. ; Kannan , K. ; Karas , BJ ; Ma, L.; et al. 2016. “Design and synthesis of a minimal bacterial genome.” Science 351 , aad6253
- Ito, Yoko, Nakagawa, Shoko, Komagata, Ayako, Masao Ikeda-Saito Yoshitsugu Shiro, and Nakamura, Hiro. 2009. “Heme-Dependent Autophosphorylation of a Heme Sensor Kinase, ChrS, from Corynebacterium Diphtheriae Reconstituted in Proteoliposomes.” FEBS Letters 583 (13): 2244–2248
- Ivens , Inge A. , Achanzar , William , Baumann , Andreas , Brändli-Baiocco , Annamaria , Cavagnaro , Joy , Dempster , Maggie , Depelchin , B. Olympe , et al. 2015. “PEGylated Biopharmaceuticals: Current Experience and Considerations for Nonclinical Development.” Toxicologic Pathology 43(7):959–983
- Iyer, Rohin K., Bowles, Paul A., Kim, Howard, and Dulgar-Tulloch, Aaron. 2018. “Industrializing Autologous Adoptive Immunotherapies: Manufacturing Advances and Challenges.” Frontiers of Medicine 5 (May): 150
- Iyer, Sukanya, Karig, David K., Norred, S. Elizabeth, Simpson, Michael L., and Doktycz, Mitchel J. 2013. “Multi-Input Regulation and Logic with T7 Promoters in Cells and Cell-Free Systems.” PloS One 8 (10): e78442
- Jaeger, L, and Chworos, A. 2006. “The architectonics of programmable RNA and DNA nanostructures.” Curr. Opin. Struct. Biol. 16, 531
- Jahn, Andreas, Vreeland, Wyatt N., Gaitan, Michael, and Locascio, Laurie E. 2004. “Controlled Vesicle Self-Assembly in Microfluidic Channels with Hydrodynamic Focusing.” Journal of the American Chemical Society 126 (9): 2674–2675
- Jermutus, L, et al. 1998. “Recent advances in producing and selecting functional proteins by using cell-free translation.” Curr. Opin. Biotechnol. 9, 534–548
- Joyce, GF. 2007. “Forty years of in vitro evolution.” Angew. Chem., Int. Ed.
- Kan, A & Joshi, NS. 2019. “Towards the directed evolution of protein materials.” MRS Commun. 9, 441–455
- Kauffman, S, and Ellington, AD. 1999. “Thinking combinatorially.” Curr. Opin. Chem. Biol. 3, 256–259
- Kim, J, et al. 2004. “Neural network computation by in vitro transcriptional circuits.” Adv. Neural Inf. Process. Syst. 17, 681–688
- Kim, Min Woo, Niidome, Takuro, and Lee, Ruda. 2019. “Glycol Chitosan-Docosahexaenoic Acid Liposomes for Drug Delivery: Synergistic Effect of Doxorubicin-Rapamycin in Drug-Resistant Breast Cancer.” Marine Drugs 17 (10)
- Kisovec, Matic, Rezelj, Saša, Knap, Primož, Mojca, Cajnko Miša, Caserman, Simon, Flašker, Ajda, Žnidaršič, Nada, et al. 2017. “Engineering a pH Responsive Pore Forming Protein.” Scientific Reports
- Kiverstein, J., Kirchhoff, M.D., and Froese, T. 2022. “The Problem of Meaning: The Free Energy Principle and Artificial Agency.” Front. Neurorobot. 16, 8447