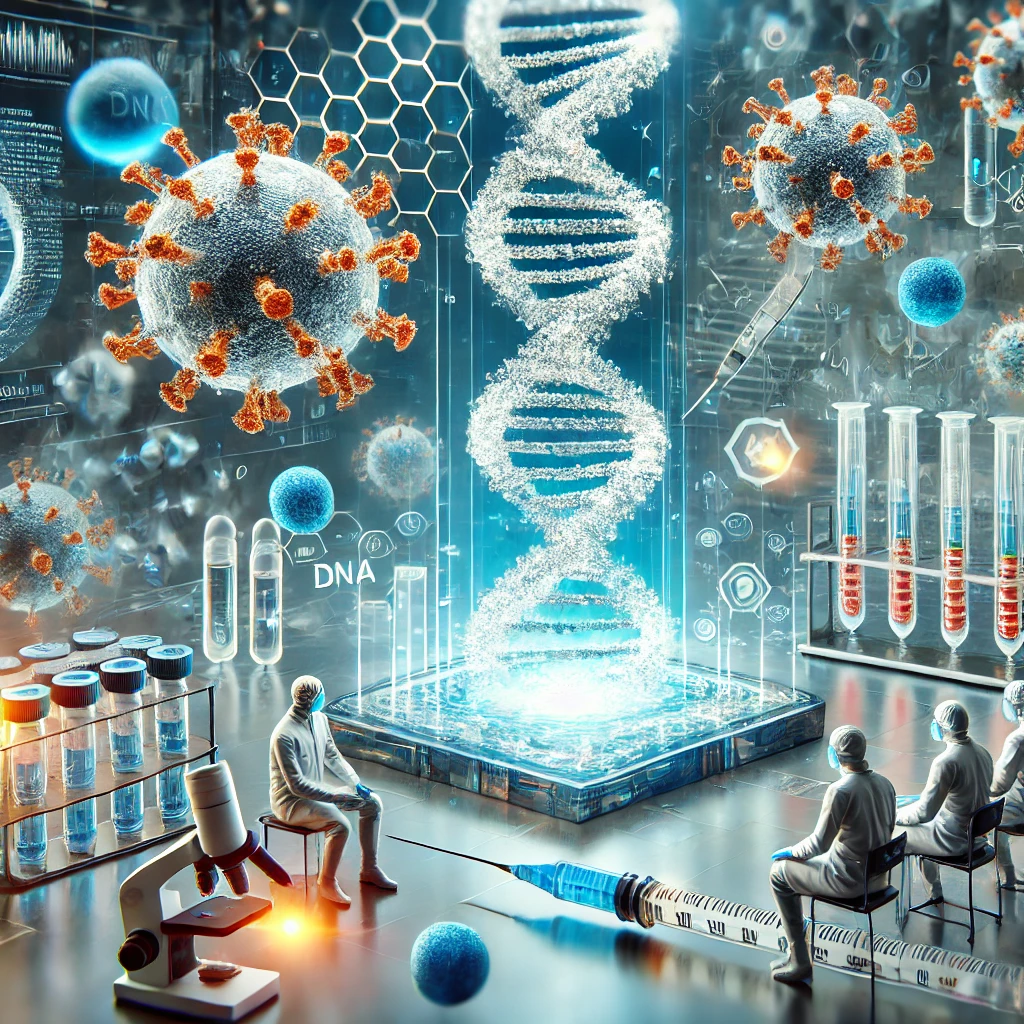
Ben ritrovati amici, e benvenuti in questo nuovo viaggio alla scoperta delle meraviglie della scienza! Oggi ci inoltreremo in un territorio affascinante e ricco di promesse, quello dell’editing genetico. Immaginate, se volessimo, di poter modificare il codice stesso della vita, di correggere gli errori impressi nel DNA, proprio come un attento editore revisiona un testo antico e prezioso. Ebbene, questa possibilità, un tempo relegata al regno della fantascienza, è oggi una realtà grazie a uno strumento rivoluzionario: CRISPR-Cas9.
Ma dove nasce questa straordinaria tecnologia? Per scoprirlo, dobbiamo immergerci nel mondo microscopico, tra batteri e archeobatteri. Questi organismi, per difendersi dagli attacchi dei virus, hanno sviluppato un sistema immunitario ingegnoso, una sorta di “archivio” di frammenti di DNA virale. Quando un virus tenta di infettare nuovamente il batterio, questo lo riconosce grazie al suo archivio e lo neutralizza. Un meccanismo affascinante, non trovate?
Ebbene, alcuni scienziati, con la loro proverbiale curiosità e intuizione, hanno compreso che questo sistema di difesa batterico poteva essere sfruttato per modificare il DNA in modo mirato. Come dei veri e propri “archeologi del genoma”, Jennifer Doudna ed Emmanuelle Charpentier, due figure chiave in questa avventura scientifica, hanno svelato il potenziale di CRISPR-Cas9, aprendo la strada a una nuova era, quella dell’editing genetico di precisione.
Ma come funziona, esattamente, questo CRISPR-Cas9? Immaginiamolo come un sofisticato bisturi molecolare, composto da due elementi principali: la proteina Cas9, che agisce come una “forbice” in grado di tagliare il DNA, e una molecola di RNA guida, che indica alla proteina Cas9 il punto esatto dove intervenire. Un meccanismo di una precisione straordinaria, che permette di intervenire sul DNA con una delicatezza e una selettività mai raggiunte prima.
Le potenzialità di CRISPR sono davvero immense, e spaziano in molteplici campi. In medicina, ad esempio, CRISPR promette di rivoluzionare la cura di malattie genetiche ereditarie, come la talassemia, la fibrosi cistica e la distrofia muscolare. Immaginate la possibilità di correggere i “refusi” genetici alla base di queste malattie, restituendo la speranza a milioni di pazienti. Ma non solo: CRISPR potrebbe anche rivelarsi un’arma formidabile nella lotta contro il cancro e le infezioni virali.
E non finisce qui! CRISPR trova applicazioni anche in agricoltura, dove può contribuire a creare colture più resistenti alle malattie e ai cambiamenti climatici, e in zootecnia, per migliorare la salute e la produttività degli animali da allevamento. Insomma, un vero e proprio “coltellino svizzero” della biologia, con un potenziale applicativo che sembra non avere limiti.
Ma come ogni grande innovazione, anche CRISPR solleva importanti interrogativi etici e sociali. La possibilità di modificare il genoma umano, ad esempio, pone questioni delicate sulla sicurezza, l’equità e il rispetto della diversità genetica. È fondamentale, quindi, che la comunità scientifica e la società nel suo complesso affrontino con responsabilità e lungimiranza le sfide poste da questa nuova tecnologia.
Il futuro di CRISPR è ancora tutto da scrivere, ma una cosa è certa: questa straordinaria scoperta ha aperto un nuovo capitolo nella storia della scienza, un capitolo ricco di promesse e di sfide. E noi, come esploratori del sapere, non possiamo che guardare con entusiasmo e curiosità a questo nuovo orizzonte, con la consapevolezza che la conoscenza è uno strumento potente, che dobbiamo utilizzare con saggezza e responsabilità per il bene dell’umanità.
Glossario
Per rendere il nostro viaggio ancora più completo, ecco un piccolo glossario dei termini che abbiamo incontrato oggi:
* DNA: il codice genetico, il “libro della vita” che contiene tutte le informazioni necessarie per lo sviluppo e il funzionamento di un organismo.
* Gene: un segmento di DNA che codifica per una specifica proteina.
* Editing genetico: la modifica mirata del DNA.
* CRISPR-Cas9: un sistema di editing genetico di precisione.
* RNA guida: la molecola che “guida” la proteina Cas9 verso il punto del DNA da modificare.
* Proteina Cas9: la “forbice molecolare” che taglia il DNA.
* Cellule germinali: le cellule riproduttive (spermatozoi e ovuli).
* Malattie ereditarie: malattie causate da alterazioni del DNA trasmesse dai genitori ai figli.
Spero che questo viaggio nel mondo di CRISPR vi sia piaciuto. Continuate a seguirmi, e insieme esploreremo le meraviglie del passato, del presente e del futuro!
Riferimenti bibliogfafici
- Abudayyeh, O. O., Gootenberg, J. S., Essletzbichler, P., Han, S., Joung, J., Belanto, J. J., … & Zhang, F. (2017). RNA targeting with CRISPR–Cas13. Nature, 550(7675), 280-284.
- Akram, F., Haq, I. U., Ali, H., & Laghari, A. T. (2018). Trends to store digital data in DNA: an overview. Molecular Biology Reports, 45(5), 1479-1490.
- Ali, G., Tariq, M. A., Shahid, K., Khan, A. M., Khan, M. S., & Abbasi, M. A. (2021). Advances in genome editing: The technology of choice for precise and efficient β-thalassemia treatment. Gene Therapy, 28(1), 6-15.
- Alipanahi, R., Safari, L., & Khanteymoori, A. (2023). CRISPR genome editing using computational approaches: A survey. Frontiers in Bioinformatics, 2, 1001131.
- Andersson, S., Antonsson, M., Elebring, M., & Lönnberg, T. (2018). Drug metabolism and pharmacokinetic strategies for oligonucleotide- and mRNA-based drug development. Drug Discovery Today, 23(9), 1733-1745.
- Anzalone, A. V., Randolph, P. B., Davis, J. R., Sousa, A. A., Koblan, L. W., Levy, J. M., … & Liu, D. R. (2019). Search-and-replace genome editing without double-strand breaks or donor DNA. Nature, 576(7785), 149-157.
- Ansori, A. N. M., Antonius, Y., Susilo, R. J. K., Hayaza, S., Kharisma, V. D., Parikesit, A. A., … & Burkov, P. (2023). Application of CRISPR-Cas9 genome editing technology in various fields: A review. Narra J, 3(2), e184.
- Bancroft, C., Bowler, T., Bloom, B., & Clelland, C. T. (2001). Long–term storage of information in DNA. Science, 293(5536), 1763-1765.
- Bansal, M., Acharya, S., Sharma, S., Singh, P., & Pandey, R. (2021). CRISPR Cas9 based genome editing in inherited retinal dystrophies. Ophthalmic Genetics, 1-10.
- Basak, A., & Sankaran, V. G. (2016). Regulation of the fetal hemoglobin silencing factor BCL11A. Annals of the New York Academy of Sciences, 1368(1), 25-30.
- Baylis, F., & Mcleod, M. (2017). First-in-human phase 1 CRISPR gene editing cancer trials: are we ready?. Current Gene Therapy, 17(4), 309-319.
- Bhatia, S., Pooja, & Yadav, S. K. (2023). CRISPR-Cas for genome editing: Classification, mechanism, designing and applications. International Journal of Biological Macromolecules, 238, 124054.
- Bhokisham, N., Laudermilch, E., Traeger, L. L., & Ghadessy, F. J. (2023). CRISPR-Cas system: The current and emerging translational landscape. Cells, 12(8), 1103.
- Bikard, D., Jiang, W., Samai, P., Hochschild, A., Zhang, F., & Marraffini, L. A. (2013). Programmable repression and activation of bacterial gene expression using an engineered CRISPR-Cas system. Nucleic Acids Research, 41(15), 7429-7437.
- Bolotin, A., Quinquis, B., Sorokin, A., & Ehrlich, S. D. (2005). Clustered regularly interspaced short palindrome repeats (CRISPRs) have spacers of extrachromosomal origin. Microbiology, 151(8), 2551-2561.
- Brennan, S., Garcia-Castaneda, M., Michelucci, A., Sabha, N., Malik, S., Groom, L., … & Shieh, J. T. C. (2019). Mouse model of severe recessive RYR1-related myopathy. Human Molecular Genetics, 28(17), 3024-3036.
- Bruegmann, T., Deecke, K., & Fladung, M. (2019). Evaluating the efficiency of gRNAs in CRISPR/Cas9 mediated genome editing in poplars. International Journal of Molecular Sciences, 20(15), 3623.
- Castillo, M. (2014). From hard drives to flash drives to DNA drives. AJNR. American Journal of Neuroradiology, 35(1), 1-2.
- Chadwick, A. C., & Musunuru, K. (2017). Treatment of dyslipidemia using CRISPR/Cas9 genome editing. Current Atherosclerosis Reports, 19(7), 32.
- Chang, A. Y. (2022). Genome engineering with CRISPR/Cas9, ZFNs, and TALENs. In CRISPR Genome Surgery in Stem Cells and Disease Tissues (pp. 39-45). Academic Press.
- Charlesworth, C. T., Deshpande, P. S., Dever, D. P., Camarena, J., Lemgart, V. T., Cromer, M. K., … & Porteus, M. H. (2019). Identification of preexisting adaptive immunity to Cas9 proteins in humans. Nature Medicine, 25(2), 249-254.
- Chatterjee, P., Jakimo, N., & Jacobson, J. M. (2018). Minimal PAM specificity of a highly similar SpCas9 ortholog. Science Advances, 4(10), eaau0766.
- Chen, H., Kazemier, H. G., de Groote, M. L., Ruiters, M. H., Xu, G. L., & Eggen, B. J. L. (2013). Induced DNA demethylation by targeting ten-eleven translocation 2 to the human ICAM-1 promoter. Nucleic Acids Research, 42(3), 1563-1574.
- Chen, H., Choi, J., & Bailey, S. (2014). Cut site selection by the two nuclease domains of the Cas9 RNA-guided endonuclease. Journal of Biological Chemistry, 289(19), 13284-13294.
- Chen, J. S., Dagdas, Y. S., Kleinstiver, B. P., … & Doudna, J. A. (2017). Enhanced proofreading governs CRISPR-Cas9 targeting accuracy. Nature, 550(7676), 407-410.
- Chen, Y., Zhang, Y. (2018). Application of the CRISPR/Cas9 system to drug resistance in breast cancer. Advanced Science, 5(11), 1700964.
- Chen, Y. C. (2021). CRISPR based genome editing and removal of human viruses. Progress in Molecular Biology and Translational Science, 179, 93-116.
- Chin, A. (2015). CRISPR-Cas9 Therapeutics: A Technology Overview. Oxford, UK: Biostars.
- Cho, S. W., Kim, S., Kim, Y., Kim, J. S., & Bae, S. (2014). Analysis of off-target effects of CRISPR/Cas-derived RNA-guided endonucleases and nickases. Genome Research, 24(1), 132-141.
- Choi, E., & Koo, T. (2021). CRISPR technologies for the treatment of Duchenne muscular dystrophy. Molecular Therapy, 29(7), 2283-2296.
- Chow, M., Chang, R., & Chan, H. K. (2021). Inhalation delivery technology for genome-editing of respiratory diseases. Advanced Drug Delivery Reviews, 168, 217-228.
- Christian, M., Cermak, T., Doyle, E. L., Schmidt, C., Zhang, F., Hummel, A., & Bogdanove, A. J. (2010). Targeting DNA double-strand breaks with TAL effector nucleases. Genetics, 186(2), 757-761.
- Collins, F. S. (2015). Statement on NIH Funding of Research Using Gene-Editing Technologies in Human Embryos. Bethesda, MD, USA: National Institutes of Health.
- Cong, L., Ran, F. A., Cox, D., Lin, S., Barretto, R., Habib, N., … & Zhang, F. (2013). Multiplex genome engineering using CRISPR/Cas systems. Science, 339(6121), 819-823.
- Cong, L., & Zhang, F. (2015). Genome engineering using CRISPR-Cas9 system. In Methods in Molecular Biology (pp. 197-217). New York, NY: Humana Press.
- Cooper, D. K., Ekser, B., Ramsoondar, J., Phelps, C., & Ayares, D. (2016). The role of genetically engineered pigs in xenotransplantation research. Journal of Pathology, 238(2), 288-299.
- Cornelis, M. C., Nugent, N. R., Amstadter, A. B., & Koenen, K. C. (2010). Genetics of post-traumatic stress disorder: review and recommendations for genome-wide association studies. Current Psychiatry Reports, 12(4), 313-326.
- Cox, D. B., Platt, R. J., & Zhang, F. (2015). Therapeutic genome editing: prospects and challenges. Nature Medicine, 21(2), 121-131.
- Cox, D. B. T., Gootenberg, J. S., Abudayyeh, O. O., Franklin, B., Kellner, M. J., Joung, J., & Zhang, F. (2017). RNA editing with CRISPR-Cas13. Science, 358(6366), 1019-1027.
- Cox, K. J., Subramanian, H., Samaniego, C. C., … & Doudna, J. A. (2019). A universal method for sensitive and cell-free detection of CRISPR-associated nucleases. Chemical Science, 10(9), 2653-2662.
- Cui, L., & Bikard, D. (2016). Consequences of Cas9 cleavage in the chromosome of Escherichia coli. Nucleic Acids Research, 44(9), 4243-4251.
- Cui, L., Vigouroux, A., Rousset, F., & Bikard, D. (2018). A CRISPRi screen in E. coli reveals sequence-specific toxicity of dCas9. Nature Communications, 9(1), 1912.
- Das, A. T., Binda, C. S., & Berkhout, B. (2019). Elimination of infectious HIV DNA by CRISPR-Cas9. Current Opinion in Virology, 38, 81-88.
- Davidson, A. R., Lu, W. T., Stanley, S. Y., Wang, J., & Sontheimer, E. J. (2020). Anti–CRISPRs: protein inhibitors of CRISPR–Cas systems. Annual Review of Biochemistry, 89, 309-332.
- Deltcheva, E., Chylinski, K., Sharma, C. M., Gonzales, K., Chao, Y., Pirzada, Z. A., … & Charpentier, E. (2011). CRISPR RNA maturation by trans-encoded small RNA and host factor RNase III. Nature, 471(7340), 602-607.
- Deng, J., Chen, M., Liu, Z., Song, Y., Sui, T., Xu, L., … & Li, Z. (2019). The disrupted balance between hair follicles and sebaceous glands in Hoxc13-ablated rabbits. The FASEB Journal, 33(1), 1226-1234.
- Dever, D. P., Bak, R. O., Reinisch, A., Camarena, J., Washington, G., Nicolas, C. T., … & Porteus, M. H. (2016). CRISPR/Cas9 β-globin gene targeting in human haematopoietic stem cells. Nature, 539(7629), 384-389.
- Ding, Q., Regan, S. N., Xia, Y., Oostrom, L. A., Cowan, C. A., & Musunuru, K. (2013). Enhanced efficiency of human pluripotent stem cell genome editing through replacing TALENs with CRISPRs. Cell Stem Cell, 12(4), 393-394.
- Ding, T., Huang, C., Liang, Z., Ma, X., & Huo, Y. X. (2020). Reversed paired-gRNA plasmid cloning strategy for efficient genome editing in Escherichia coli. Microbial Cell Factories, 19(1), 63.
- Doudna, J. A., & Charpentier, E. (2014). The new frontier of genome engineering with CRISPR-Cas9. Science, 346(6213), 1258096.
- Drost, J., van Jaarsveld, R. H., Ponsioen, B., Zimberlin, C., van Boxtel, R., Buijs, A., … & Clevers, H. (2015). Sequential cancer mutations in cultured human intestinal stem cells. Nature, 521(7550), 43-47.
- Duan, J. Z., Lu, G., Xie, Z., Chan, K. W., Zhang, X., Zhu, J., … & Chen, S. (2014). Genome-wide identification of CRISPR/Cas9 off-targets in human genome. Cell Research, 24(8), 1009-1012.
- Egorova, T. V., Zotova, E. D., Reshetov, D. A., Polikarpova, A. V., Vassilieva, S. G., Vlodavets, D. V., … & Zakharova, L. A. (2019). CRISPR/Cas9-generated mouse model of Duchenne muscular dystrophy recapitulating a newly identified large 430 kb deletion in the human DMD gene. Disease Models & Mechanisms, 12(7), dmm038513.
- Eid, A., Alshareef, S., & Mahfouz, M. M. (2018). CRISPR base editors: genome editing without double-stranded breaks. Biochemical Journal, 475(12), 1955-1964.
- Esvelt, K. M., & Wang, H. H. (2013). Genome‐scale engineering for systems and synthetic biology. Molecular Systems Biology, 9(1), 641.
- Fan, Z., Perisse, I. V., Cotton, C. U., Regouski, M., Meng, Q., Domb, C., … & Fiegel, V. (2018). A sheep model of cystic fibrosis generated by CRISPR/Cas9 disruption of the CFTR gene. JCI Insight, 3(20).
- Fellmann, C., Gowen, B. G., Lin, P. C., Doudna, J. A., & Corn, J. E. (2015). Cornerstones of CRISPR-Cas9 Technology. Cell Stem Cell, 16(3), 229-242.
- Ferdosi, S. R., Ewaisha, R., Moghadam, F., … & Fadel, R. M. (2019). Multifunctional CRISPR-Cas9 with engineered immunosilenced human T cell epitopes. Nature Communications, 10(1), 1842.
- Firth, A. L., Menon, T., Parker, G. S., Qualls, S. J., Lewis, B. M., Ke, E., … & Wyman, A. E. (2015). Functional Gene Correction for Cystic Fibrosis in Lung Epithelial Cells Generated from Patient iPSCs. Cell Reports, 12(9), 1385-1390.
- Fry, L. E., McClements, M. E., & MacLaren, R. E. (2021). Analysis of pathogenic variants correctable with CRISPR base editing among patients with recessive inherited retinal degeneration. JAMA Ophthalmology, 139(3), 319-328.
- Fu, Y., Sander, J. D., Reyon, D., Cascio, V. M., & Joung, J. K. (2014). Improving CRISPR-Cas nuclease specificity using truncated guide RNAs. Nature Biotechnology, 32(3), 279-284.
- Fuchs, G., Petrov, A. N., Marceau, C. D., Popov, L. M., Chen, J., & Kieft, J. S. (2015). Kinetic pathway of 40S ribosomal subunit recruitment to hepatitis C virus internal ribosome entry site. Proceedings of the National Academy of Sciences, 112(10), 319-325.
- Gallego, C., Gonçalves, M., & Wijnholds, J. (2020). Novel therapeutic approaches for the treatment of retinal degenerative diseases: Focus on CRISPR/Cas-based gene editing. Frontiers in Neuroscience, 14, 838.
- Gantz, V. M., Jasinskiene, N., Tatarenkova, O., Fazekas, A., Macias, V. M., … & James, A. A. (2015). Highly efficient Cas9-mediated gene drive for population modification of the malaria vector mosquito Anopheles stephensi. Proceedings of the National Academy of Sciences, 112(49), E6736-E6743.
- Gao, X., Tao, Y., Lamas, V., Huang, M., Yeh, W. H., Pan, B., … & Liu, D. R. (2018). Treatment of autosomal dominant hearing loss by in vivo delivery of genome editing agents. Nature, 553(7687), 217-221.
- Gao, Z., Fan, M., Das, A. T., … & Berkhout, B. (2020). Extinction of all infectious HIV in cell culture by the CRISPR-Cas12a system with only a single crRNA. Nucleic Acids Research, 48(10), 5527-5539.
- Gilbert, L. A., Larson, M. H., Morsut, L., Liu, Z., Brar, G. A., … & Qi, L. S. (2013). CRISPR-mediated modular RNA-guided regulation of transcription in eukaryotes. Cell, 154(2), 442-451.
- Gillings, M. R., Hilbert, M., & Kemp, D. J. (2016). Information in the biosphere: biological and digital worlds. Trends in Ecology & Evolution, 31(3), 180-189.
- Gootenberg, J. S., Abudayyeh, O. O., Essletzbichler, P., … & Zhang, F. (2018). Multiplexed and portable nucleic acid detection platform with Cas13, Cas12a, and Csm6. Science, 360(6387), 439-444.
- Gootenberg, J. S., Abudayyeh, O. O., Kellner, M. J., Joung, J., Collins, J. J., & Zhang, F. (2017). Nucleic Acid Detection with CRISPR-Cas13a/C2c2. Science, 356(6336), 438-442.
- Gracheva, E. O., Ingolia, N. T., Kelly, Y. M., Cordero-Morales, J. F., Hollopeter, G., Chesler, A. T., … & Julius, D. (2010). Molecular basis of infrared detection by snakes. Nature, 464(7291), 1006-1011.
- Granados-Riveron, J. T., & Aquino-Jarquin, G. (2018). CRISPR-Cas13 precision transcriptome engineering in cancer. Cancer Research, 78(15), 4107-4113.
- Greely, H. T. (2019). Human germline genome editing: an assessment. CRISPR Journal, 2(5), 253-265.
- Guo, D., Liu, H., Gao, G., Liu, G., Dong, W., Li, X., … & Zhao, Y. (2017). Creating a patient carried Men1 gene point mutation on wild type iPSCs locus mediated by CRISPR-Cas9 and ssODN. Stem Cell Research, 18, 67-69.
- Guo, M., Zhang, X., Liu, J., … & Wang, S. (2020). OsProDH negatively regulates thermotolerance in rice by modulating proline metabolism and reactive oxygen species scavenging. Rice, 13(1), 1-5.
- Hale, C. R., Zhao, P., Olson, S., Duff, M., & Guthrie, C. R. (2009). RNA-guided RNA cleavage by a CRISPR RNA-Cas protein complex. Cell, 139(5), 945-956.
- Hammer, M. J., Eckardt, P., & Barton-Burke, M. (2016). Informed consent: a clinical trials perspective. Oncology Nursing Forum, 43(6), 694-696.
- Harden, M. E., & Munger, K. (2017). Human papillomavirus molecular biology. Mutation Research/Reviews in Mutation Research, 772, 3-12.
- Hartong, D. T., Berson, E. L., & Dryja, T. P. (2006). Retinitis pigmentosa. The Lancet, 368(9549), 1795-1809.
- Hess, G. T., Tycko, J., Yao, D., & Bassik, M. C. (2017). Methods and applications of CRISPR-mediated base editing in eukaryotic genomes. Molecular Cell, 68(1), 26-43.
- High, K. A., & Roncarolo, M. G. (2019). Gene Therapy. New England Journal of Medicine, 381(5), 455-464.
- Hille, F., Richter, H., Wong, S. P., Bratovič, M., Ressel, S., & Charpentier, E. (2018). The biology of CRISPR-Cas: backward and forward. Cell, 172(6), 1239-1259.
- Hirsch, M. L., Agbandje-McKenna, M., & Samulski, R. J. (2010). Little vector, big gene transduction: fragmented genome reassembly of adeno-associated virus. Molecular Therapy, 18(1), 6-8.
- Hirsch, M. L., Wolf, S. J., & Samulski, R. J. (2016). Delivering Transgenic DNA Exceeding the Carrying Capacity of AAV Vectors. Methods in Molecular Biology, 1382, 21-39.
- Hosseini Far, H., Patria, Y. N., Motazedian, A., Elefanty, A. G., Stanley, E. G., Lamande, S. R., … & O’Brien, T. J. (2019). Generation of a heterozygous COL1A1 (c.3969_3970insT) osteogenesis imperfecta mutation human iPSC line, MCRIi001-A-1, using CRISPR/Cas9 editing. Stem Cell Research, 37, 101449.
- Hsu, P. D., Scott, D. A., Weinstein, J. A., Ran, F. A., Konermann, S., Agarwala, V., … & Zhang, F. (2013). DNA targeting specificity of RNA-guided Cas9 nucleases. Nature Biotechnology, 31(9), 827-832.
- Hu, Z., Yu, L., Zhu, D., Ding, W., Wang, X., Zhang, C., … & Zhao, R. (2014). Disruption of HPV16-E7 by CRISPR/Cas system induces apoptosis and growth inhibition in HPV16 positive human cervical cancer cells. BioMed Research International, 2014, 612823.
- Huang, X., Wang, Y., Yan, W., Smith, C., Ye, Z., Wang, J., … & Yang, S. (2015). Production of gene-corrected adult beta globin protein in human erythrocytes differentiated from patient iPSCs after genome editing of the sickle point mutation. Stem Cells, 33(5), 1470-1479.
- Hui, S-W. (2008). Overview of drug delivery and alternative methods to electroporation bt – electroporation protocols: preclinical and clinical gene medicine. In S Li (Ed.), Electroporation Protocols Methods in Molecular Biology, 91-107. Totowa, NJ: Humana Press.
- Hynes, R. O., Coller, B. S., & Porteus, M. (2017). Toward responsible human genome editing. JAMA, 317(18), 1829-1830.
- Ihry, R. J., Worringer, K. A., Salick, M. R., Frias, E., Ho, D., Theriault, K., … & Hemann, M. T. (2018). p53 inhibits CRISPR–Cas9 engineering in human pluripotent stem cells. Nature Medicine, 24(7), 939-946.
- Ilahibaks, N. F., Hulsbos, M. J., Lei, Z., Geijsen, N., & Hatzimanikatis, V. (2023). Enabling precision medicine with CRISPR-Cas genome editing technology: A translational perspective. Advances in Experimental Medicine and Biology, 1396, 315-339.
- Jasanoff, S., & Hurlbut, J. B. (2018). A global observatory for gene editing. Nature, 555(7697), 435-437.
- Jarrett, K. E., Lee, C., De Giorgi, M., Hurley, A., Gillard, B. K., Doerfler, A. M., … & Chinnaiyan, A. M. (2018). Somatic Editing of Ldlr With Adeno-Associated Viral-CRISPR Is an Efficient Tool for Atherosclerosis Research. Arteriosclerosis, Thrombosis, and Vascular Biology, 38(9), 1997-2006.
- Jia, H. (2019). China approves ethics advisory group after CRISPR-babies scandal. Nature.
- Jiang, F., & Doudna, J. A. (2017). CRISPR-Cas9 structures and mechanisms. Annual Review of Biophysics, 46, 505-529.
- Jiang, W., Bikard, D., Cox, D., Zhang, F., & Marraffini, L. A. (2013). RNA-guided editing of bacterial genomes using CRISPR-Cas systems. Nature Biotechnology, 31(3), 233-239.
- Jiang, W., Zhou, H., Bi, H., Fromm, M., Yang, B., & Weeks, D. P. (2013). Demonstration of CRISPR/Cas9/sgRNA-mediated targeted gene modification in Arabidopsis, tobacco, sorghum and rice. Nucleic Acids Research, 41(20), e188.
- Jinek, M., East, A., Cheng, A., Lin, S., Ma, E., & Doudna, J. (2013). RNA–programmed genome editing in human cells. eLife, 2, e00471.
- Jing, R., Corbett, J. L., Cai, J., … & Mostoslavsky, R. (2018). A screening using iPSCs-derived hepatocytes reveals NAD+ as a potential treatment for mtDNA depletion syndrome. Cell Reports, 25(6), 1469-1484.
- Kaul, T., Raman, N. M., Eswaran, M., … & Srivastava, A. K. (2019). Data mining by pluralistic approach on CRISPR gene editing in plants. Frontiers in Plant Science, 10, 801.
- Kalds, P., Gao, Y., Zhou, S., Cai, B., & Chen, Y. (2020). Redesigning small ruminant genomes with CRISPR toolkit: overview and perspectives. Theriogenology, 147, 25-33.
- Kang, J. T., Ryu, J., Cho, B., Lee, S., Kim, J. H., Kim, M., … & Kim, J. J. (2016). Generation ofRUNX3knock-out pigs using CRISPR/Cas9-mediated gene targeting. Reproduction in Domestic Animals, 51(6), 970-978.
- Kennedy, E. M., Cullen, B. R. (2017). Gene Editing: A New Tool for Viral Disease. Annual Review of Medicine, 68(1), 401-411.
- Kennedy, E. M., Kornepati, A. V., Goldstein, M., Bogerd, H. P., Poling, B. C., Whisnant, R. E., … & Cullen, B. R. (2014). Inactivation of the human papillomavirus E6 or E7 gene in cervical carcinoma cells by using a bacterial CRISPR/Cas RNA-guided endonuclease. Journal of Virology, 88(20), 11965-11972.
- Kim, H. K., Lee, S., Kim, Y., Park, J., Min, S., Choi, J. W., … & Kim, J. S. (2020). High-throughput analysis of the activities of xCas9, SpCas9-NG and SpCas9 at matched and mismatched target sequences in human cells. Nature Biomedical Engineering, 4(2), 111-124.
- Kim, S., Kim, D., Cho, S. W., & Kim, J. S. (2014). Highly efficient RNA-guided genome editing in human cells via delivery of purified Cas9 ribonucleoproteins. Genome Research, 24(6), 1012